We study quantum and condensed matter physics in nano-electronic devices. We want to uncover novel fundamental physical phenomena and help testing and developing physical theories. However, our research also belongs to the emerging discipline of quantum engineering, which aims to develop novel functional devices based on quantum principles. In particular, we develop and investigate novel types of qubits with the aim of impacting the development of a future quantum computer.
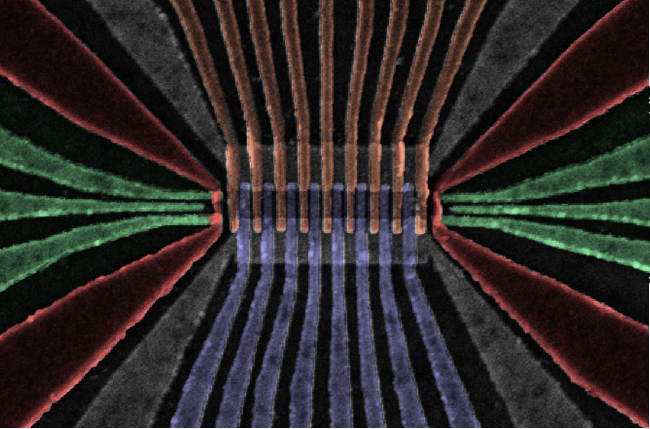
Spin qubits in Germanium quantum wells
Germanium quantum wells are at the forefront of spin qubit research. The strong confinement and compressive strain in the quantum well significantly alter the band structure, with detailed consequences on the physics of hole spins largely unknown. We aim to understand and control the physics of these quantum wells. By focusing on small scale hole spin qubit devices and studying properties such as g-factor anisotropy, spin-orbit interaction anisotropy, anisotropy of qubit coherence, and their electric field tunability we aim to understand the often complex and intricate physics underpinning this. Together with our collaborators at IHP who grow and optimise the heterostructure, we will explore novel germanium based heterostructures. Here the aim is to optimise spin qubit performance and add novel functionality to spin qubit devices. Our ultimate goal is to provide a thorough, physics based assessment of the platform’s potential for scaling up spin qubit based quantum computing.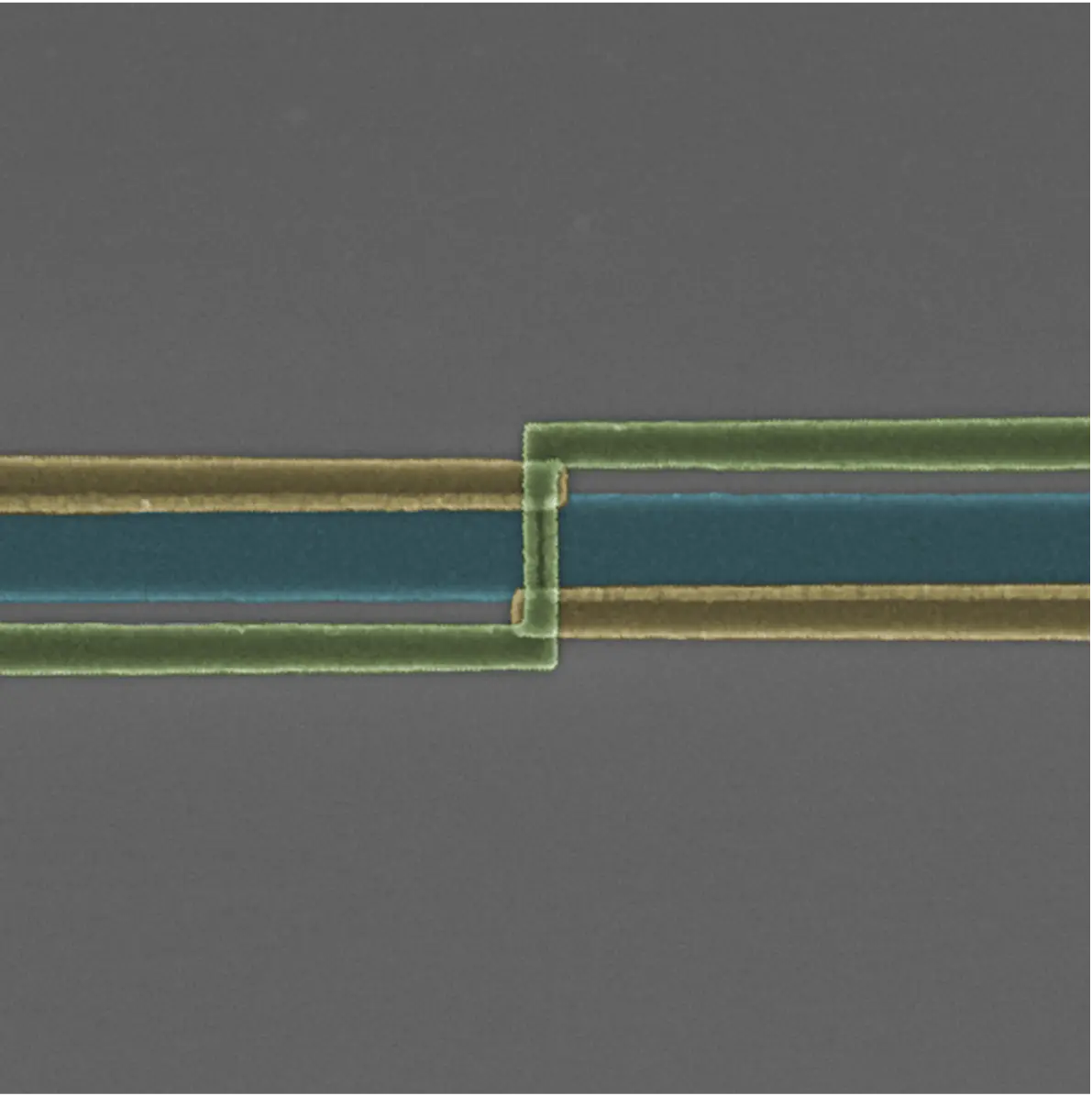
Hybrid superconducting-semiconducting quantum devices
Superconductivity continues to surprise us even after more than a hundred years. Bringing a superconductor in direct contact with a semiconductor can result in a nano-electronic device with hybrid characteristics of the parent materials. This principle is at the core of truly fascinating physics such as topological superconductivity. But well-known phenomena like the Josephson effect can become exotic too. The current-phase relationship might become electric and magnetic field controllable. Integrating such more exotic Josephson junctions in devices suitable for microwave spectroscopy or transmon style qubit devices opens up novel avenues to probe the underlying physics of Andreev Bound States. We pursue this primarily in Germanium quantum wells. Superconducting contact optimization is a crucial aspect of this research. By understanding and controlling the physics of hybrid superconductor-semiconductor hybrid devices we will assess their potential for optimising quantum devices such as the transmon qubit.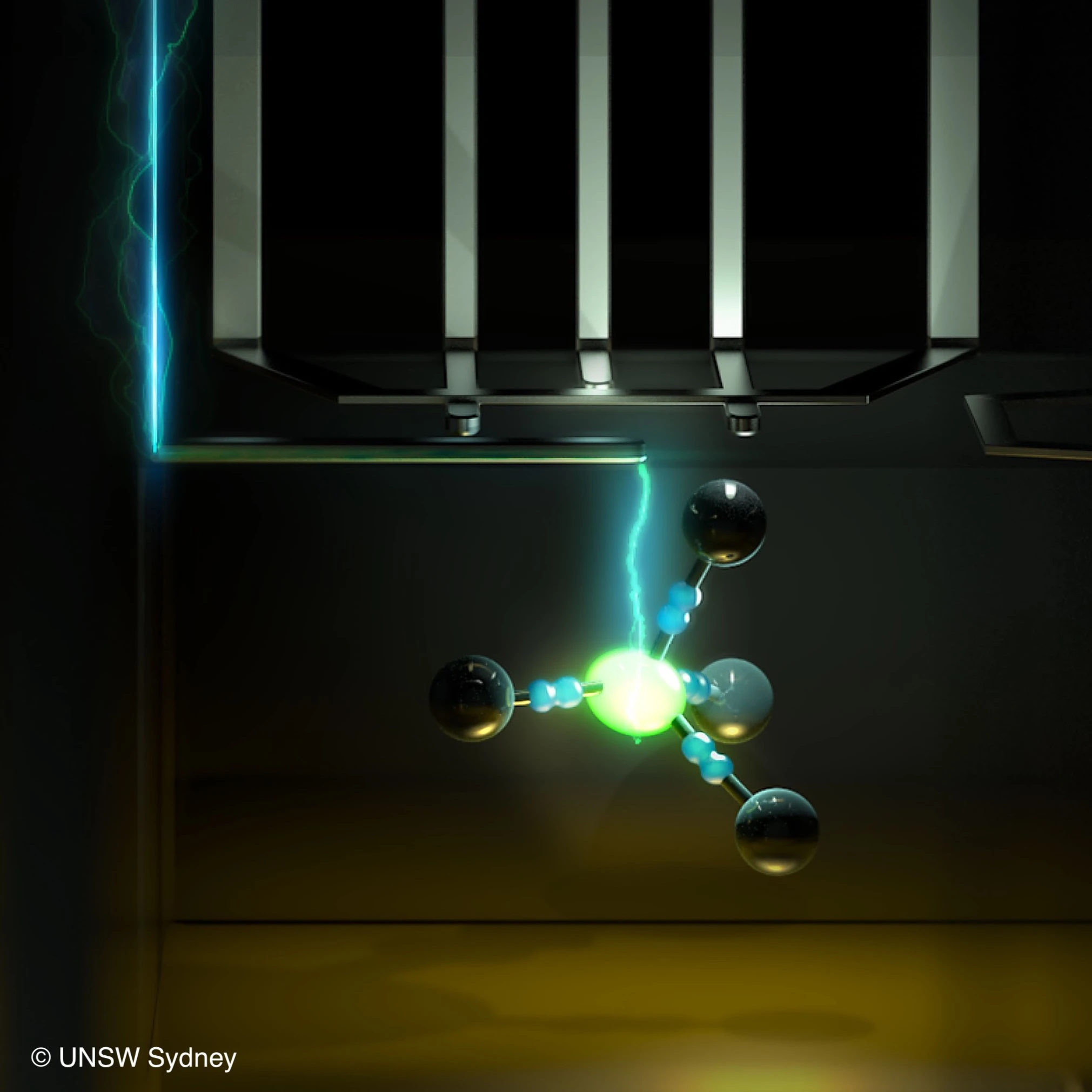
Quantum foundations
A nuclear spin embedded as a single defect in silicon or germanium is a fantastic model object to test and explore more fundamental aspects of quantum theory. To this end, the silicon or germanium host lattice needs to be isotopically purified to remove spurious background nuclear spins. This results in extremely coherent single quantum systems in the solid state. By using a single nucleus with a large nuclear spin of e.g. 7/2 or 9/2, non-linear interactions in the single particle Hamiltonian may become significant, leading to fascinating non-linear quantum dynamics. Using electronic or mechanical degrees of freedom such a nuclear spin can be accessed in nanoscale devices. We explore candidate systems, initially through modelling, and subsequently in experiment, that might implement non-linear single particle Hamiltonians of sufficient complexity. Topics we aim to research include scrambling, chaoticity and decoherence.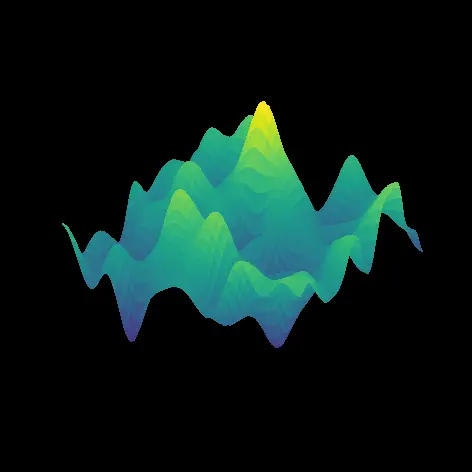